Degradation: Soil, Organic Carbon Content, Overuse & Climate are Interconnected
The quality of soil is deterionrating, it is losing organic carbon - due to climate change and unsustainable agriculture. What is the potential for regeneration of deteriorated soil?

Soil – also known as geo-derma or skin of the Earth – is a 4-dimensional body (length, width, depth and time) comprising a layer of biogeochemically transformed parent rock and sediments with a depth of about one meter. Soil produces more than 95% of all food for humans and is a habitat for about two-thirds of all biodiversity (prior estimates were one-fourth of all biodiversity). However, this finite and precious resource is being degraded, leading to a reduction of its capacity to provide critical ecosystem services for humans and nature by natural and planetary processes. One third of all soils of ice-free land area – 39.3 million (M) km2 out of 131 Mkm2 – are affected by human-induced degradation. Thus, soil degradation should be considered the 10th Earth system process in the planetary boundary framework.
Soil degradation is both affected by and contributes to anthropogenic climate change. The extent and severity of global soil degradation has strong adverse impacts on the cycling of soil organic carbon (SOC) and nutrients such as nitrogen, phosphorus and sulphur; on the quality and renewability of water; on the quality and quantity of food production; and on global peace and political stability.
Dominant soil degradation processes include physical processes such as crusting, compaction, drought-flood syndrome and accelerated erosion by water and wind; chemical processes such as salinization, acidification, nutrient depletion, pollution and contamination; biological processes such as a decline in soil organic matter content or a reduction in activity and species diversity of soil biota; and ecological processes such as alterations in soil temperature and moisture regimes, drastic shifts in flora and fauna distribution, disruptions in ecosystems, and a decline in productive capacity.
Large losses of SOC upon conversion of forestlands, grasslands, and wetlands to agricultural lands, combined with the conversion of about 1 M km2 to croplands between 1960 and 2020, have contributed substantially to anthropogenic climate change. Decline in SOC content may range from 15 to 25 % upon conversion of natural land to cropland or grazing land. In contrast, conversion of cropland or grazing land to forest or grassland may increase SOC content from 25 to 60% depending on the degree of SOC depletion, climate and soil type. However, reaching a new equilibrium of SOC stock may take up to 80 years after land-use change.
Losses due to conversion
Large losses of SOC upon conversion of forestlands, grasslands, and wetlands to agricultural lands, combined with the conversion of about 1 M km2 to croplands between 1960 and 2020, have contributed substantially to anthropogenic climate change. Decline in SOC content may range from 15 to 25 % upon conversion of natural land to cropland or grazing land. In contrast, conversion of cropland or grazing land to forest or grassland may increase SOC content from 25 to 60% depending on the degree of SOC depletion, climate and soil type. However, reaching a new equilibrium of SOC stock may take up to 80 years after land-use change.
Climate change can further decrease the SOC stocks: directly via an increase in temperature, CO2 fertilization, extreme events, and changes in both the amount and the pattern of precipitation; and indirectly, for instance due to wildfires (the occurrence of forest fires has doubled in the last 40 years), as well as due to the drought/flood syndrome and an increased frequency of extreme events.
About 30% of the world’s ice free land (39.3 M km2) is moderately to highly degraded, and the global magnitude of water erosion may be aggravated by current and future climate change. Soils are compaction-stressed in the Global North, erosion-stressed in the Global South, and SOC-stressed globally in both North and South.
An optimal level of SOC in the root zone of crop land ranges from 1.0 to 1.5 % for agricultural soils of the tropics and from 1.5 to 2.0 % for those of temperate climates. A large proportion of agricultural soils, especially those managed by 500 to 600 million small land holders of developing countries, may have SOC content as low as 0.1% in the root zone with a depth of up to 30 cm. Soil degradation, aggravated by the depletion of SOC content in the root zone, can reduce agronomic production especially on low-input systems.
Yet, achieving global net zero emissions by 2050 is critical to limiting global warming to 1.5 °C by the end of 21st Century. In conjunction with the widespread use of non-carbon fuel sources, the preservation and restoration of SOC can contribute strongly to the potential of natural climate solutions. Indeed, there is a large potential for carbon sequestration in degraded and depleted agricultural soils of the world.
Carbon losses due to increased usage
Globally, a net average decrease of about 60 metric tons of carbon per km2 per year has been reported with a total loss of 3.1 billion (B) tons of carbon from an area of 2 Mkm2 over about 25 years. It is estimated that between 2001 and 2015, 79% of countries worldwide have been affected by a net decline of SOC. The declining trend in SOC content in agro-ecosystems could have strong adverse effects on providing critical ecosystem services essential to human wellbeing and nature conservation including poor nutritional quality of food grown on these soils.
Soil Compaction
The optimum bulk density of soil in the rootzone is 1.2 to 1.3 tons per m3 (t/m3). However, traffic by heavy farm machinery can compact the soil to a bulk density of 1.4 to 1.6 t/m3. Excessive soil compaction restricts root penetration, water infiltration and retention, and gaseous exchange.
Soil compaction can also lead to anaerobiosis and aggravate emission of greenhouse gases with high global warming potential such as methane and nitrous oxide. Through restricted root growth and a decrease in the soil volume that can be accessed for uptake of water and nutrients, soil compaction can have drastic adverse effects on agronomic productivity, nutritional quality and amount of food produced, filtration and renewability of water, and on the efficiency of inputs such as fertilizer or irrigation.
Loss of Arable Land
Soil degradation by diverse processes (physical, chemical, biological, ecological) can lead to a loss of arable land. Most soil degradation processes are set in motion by a decline in soil structure caused by the depletion of SOC content. Therefore, soil restorative processes must begin with the adoption of land use and soil management practices which create a positive carbon budget in the soil or ecosystem and improve soil structure and the attendant activity and species diversity of soil biota.
Soil Organic Carbon Content and Agronomic Yield
With a human population of eight billion which is destined to exceed 11.4 B by 2100, soil degradation is a global issue which is a risk multiplier in aggravating food and nutritional insecurity. For example, severe depletion of SOC content in the rootzone can reduce agronomic yield and its nutritional quality.
In addition to plow-based tillage and unbalanced use of nutrients (such as increased use of nitrogen and reduced use of phosphorus, potassium, calcium, magnesium and micronutrients) and flood-based irrigation, burning of crop residues also has adverse effects on SOC content. Residue burning is widely practiced globally. For instance, the burning of rice straw is widespread in South Asia because of the urgency to seed wheat and the lack of appropriate technology to adopt conservation agriculture that facilitates wheat seeding through the mulch of rice straw.
Figure 1
The benefits of restoring SOC content in depleted soils include improved yields, increased resilience and decreased inputs of fertilizer and irrigation water. The critical role of SOC in yield outcomes goes beyond nutrient availability because of its potential to represent a wide range of soil functions such as improved soil structure, better aeration, favorable soil moisture and temperature regimes, high activity and diversity of soil biota, high use efficiency of inputs and inherent resources. With two-thirds of global maize and wheat land being at low to extremely low levels, there is a significant opportunity to increase SOC content (see Table 1) to reduce inputs of fertilizers and water and globally close the yield gap.
Risks of Global Decline in Soil Organic Carbon Content with Anthropogenic Climate Change
Over and above the adverse effects of agronomic practices (e.g., plowing, residue removal or burning, excessive grazing, drainage of wetlands), the current and projected climate change may also deplete SOC content in global agro-ecosystems.
An optimal level of SOC content provides farmers with a natural insurance against climate change through a gain in yield stability and more resilient production. Indeed, higher SOC content increases yield gains from favorable conditions and reduces yield losses due to adverse weather events.
However, agricultural management practices which restore SOC stocks, thus contributing to climate change mitigation and adaptation, may come at the cost of foregone yields for the farmer in the short term. Thus, to reduce risks of soil degradation and make crop production more resilient to climate change, there is a need for agricultural policies that address the public benefits of soil conservation and restoration. SOC accumulation in the short term is possible with abundant application of organic biomass, but the strategy may decrease yields over the short term. Yet, increasing SOC combined with optimal nitrogen management can lead to low chemical nitrogen input and higher grain yields.
Carbon Farming
Increasing SOC stocks by adopting restorative land use and site-specific best management practices which create positive ecosystem carbon budgets can also create another income stream for land managers. The process of creating an income stream through sequestration of atmospheric CO2 into terrestrial carbon pools (soil, trees, wetlands, coastal ecosystems) leads to “growing carbon” in land-based sinks as a new crop with both economic and ecological benefits. The societal cost of one carbon credit (1 metric ton of CO2 equivalent) is US $50 based on the inherent value of carbon stored in land-based sinks. Thus, farmers should be rewarded for conversion to restorative land use and adoption of regenerative agricultural practices through payments for ecosystem services.
Returning Land to Nature
To meet the Paris Agreement on limiting the mean global temperature increase to 1.5 °C by 2100 requires a cumulative decrease in CO2 emissions of around 3000 gigatons CO2. Whereas the capacity of agricultural soils to sequester carbon may be limited in helping to achieve the cumulative decrease to the desired level by 2100, returning land to nature may be a viable alternative. Almost 38% of Earth’s ice-free land area (50 Mkm2) is used for agriculture, of which 75% is allocated to raising animals. Furthermore, 40% of all agricultural land (20 Mkm2) is degraded by at least one of several processes. Additionally, 70% of global fresh water is used for irrigation. As much as 200 Mt of chemical fertilizers are used on agricultural land annually, and thus, 30-35% of global greenhouse gas emissions are contributed by agriculture.
Yet one in eight persons is food-insecure and two or three in eight are malnourished and prone to hidden hunger. At the same time, more than 1 Bt of food grains (about 30% of the total) are wasted and reach no stomach – either human or animal. The wastage may be much more (40 - 60%) in fresh fruits and vegetables. Business as usual is not acceptable. There is an urgent need for a radical transformation of agricultural and food systems.
Global Strategy
The strategy must be to produce more from less: less land, less water, less fertilizer, less energy input. The most natural solution can be returning some land back to nature. A possible scenario of returning land and water to nature is outlined in Table 1. By 2100, half of the current cropland (750 Mha) and 60% of grazing land area (2.25 Bha) must be returned to nature. Whereas the land area under irrigation must be increased from 350 Mha at present to 750 Mha by 2100, the water used for irrigation must be decreased from 3150 km3 at present to 1000 km3 by 2100 through the use of drip fertigation systems, i.e. combined fertilization and irrigation. Translating known science into action by returning land and water to nature and restoring degraded lands needs policies which are pro-nature, pro-farmers and pro-agriculture.
Table 1. A scenario timetable for humanity to return land and other resources to nature and rough estimates of carbon sequestration in land spared.
Resource Use | Units | 2020 | 2030 | 2050 | 2100 |
Fertilizer | Mt/yr. | 200 | 150 | 100 | 50 |
Irrigated Area | Mha | 350 | 400 | 450 | 500 |
Cropland Area | Mha | 1500 | 1400 | 1000 | 750 |
Conservation Agriculture | Mha | 200 | 250 | 500 | 750 |
Grazing/Rangeland | Mha | 3700 | 3500 | 2500 | 1500 |
Water use of Agriculture | km3 | 3150 | 3000 | 2000 | 1000 |
Global Cereal Yield | ton/ha | 4.00 | 4.5 | 6.00 | 8.00 |
Carbon Sequestration (Soil and Trees) | MtC/yr. | 100 1 | (87 + 105 + 300) = 492 2 | (125 + 425 + 1700) = 2250 3 | (150 + 590 + 2950) = 3690 4 |
1 – Soil carbon sequestration in 200 Million hectares (Mha) of Conservation Agriculture (CA) @ 0.5 tons of Carbon per hectare per year (tC/ha.yr)
2 – 250 Mha of CA @ 0.35 tC/ha.yr, 300 Mha of land returned to nature @ 0.35 tC/ha.yr in soil and 1 tC/ha.yr in vegetation biomass.
3 – 500 Mha of CA @ 0.25 tC/ha.yr, 1700 Mha of land returned to nature @ 0.25 tC/ha.yr in soil, and 1 tC/ha.yr in vegetation biomass.
4 – 750 Mha under CA @ 0.2 tC/ha.yr, 2950 Mha land returned to nature @ 0.2 tC/ha.yr in soil and 1 tC/ha.yr in vegetation biomass. The rate of SOC sequestration has progressively been decreased. But the rate of C sequestration in biomass is assured at 1 tC/ha.yr
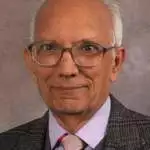